Two recent papers co-authored by Luis Valentin-Alvarado, PhD ’23 Microbiology, offer new insight into the distribution and function of Asgard archaea, a group of microorganisms that share a common ancestor with eukaryotes—including humans.
Researchers first discovered Asgard archaea in extreme environments like deep-sea hydrothermal vents and hot springs, but subsequent studies have confirmed the presence of microorganisms in marine, freshwater, and anaerobic environments. As a graduate student, Valentin-Alvarado worked with Jillian Banfield, a professor in the Departments of Environmental Science, Policy, and Management and Earth and Planetary Sciences, to sample soil from California wetlands and sequence their microbial communities.
Valentin-Alvarado is co-first author of a Cell study published in June that found that certain archaea possess FeFe hydrogenases involved in hydrogen production—a function thought to only exist in bacteria and eukaryotes. A new study published last Wednesday in Nature Communications introduces Atabeyarchaeia, a novel archaeal family discovered and named by Valentin-Alvarado that may interact with the methane-producing organisms in the soil.
We spoke to Valentin-Alvarado about his research, the significance behind the name Atabeyarchaeia, and what these findings could mean for addressing climate change.
The conversation has been edited for length and clarity.
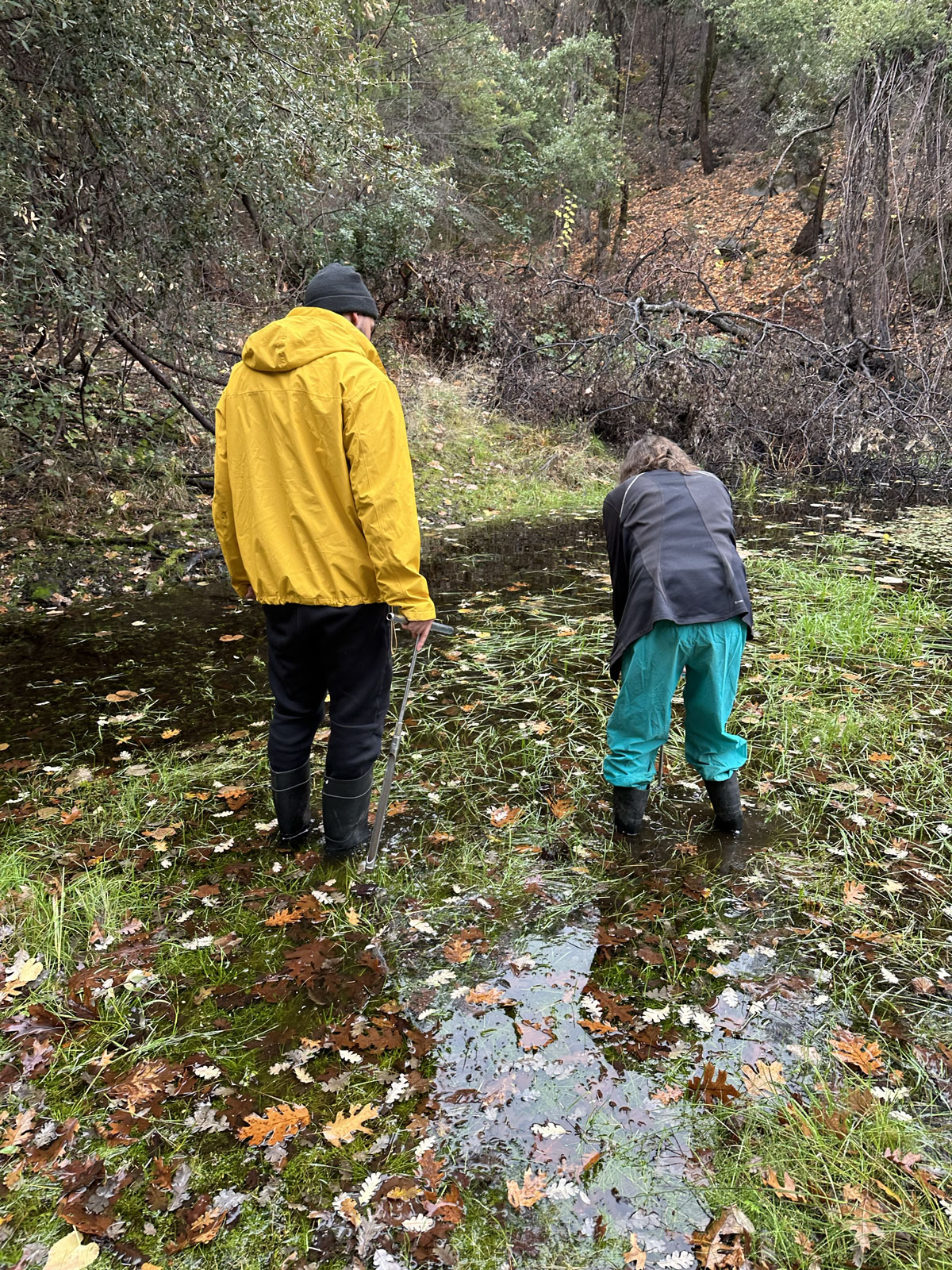
What can you tell us about your research?
My research focuses on genome-resolved metagenomics, an approach to understanding microbial communities in various environments. I like to think of it as solving a complex puzzle. We take environmental samples, sequence the DNA present, and then piece together the genomes of individual microorganisms within these communities. This allows us to gain insights into the genetic potential and metabolic capabilities of microbes that we often can't culture in the lab.
A significant part of my work centers on archaea; people usually talk about bacteria in anaerobic environments, which have little or no free oxygen. Archaea is another group of organisms that are equally important in such environments, but they’re usually dismissed because people think of archaea as only being found in extreme places like Yellowstone hot springs or deep in the ocean. That’s not true—they’re everywhere.
Our Cell paper looks across environments for archaeal genomes that encode—or potentially encode—hydrogenases. I mined the Banfield lab’s genome database to try to find novel archaeal hydrogenases. We found a really peculiar group of hydrogenases—an iron-iron hydrogenase—that, before this discovery, were widely believed to only be present in bacteria and eukaryotes. We found that they're also present in archaea. It’s quite a big deal because it made us rethink the evolution of these ancient enzymes, and is something that has an important economic aspect—especially as hydrogen gets more attention as a potential fuel source. This was a collaboration with Chris Greening, a professor of microbiology at Monash University in Australia, on validating and characterizing these archaea.
We also found this very weird archaeal group that hinted toward the possibility of being part of a new candidate group. We usually run a screening profile on archaea, and when we did, it didn’t return a hit to something we knew we had. Jill said that it was novel, so I did the phylogenetic reconstruction and started investigating the genome. What I found, which is the focus of the Nature Communications paper, was two new groups of Asgard archaea.
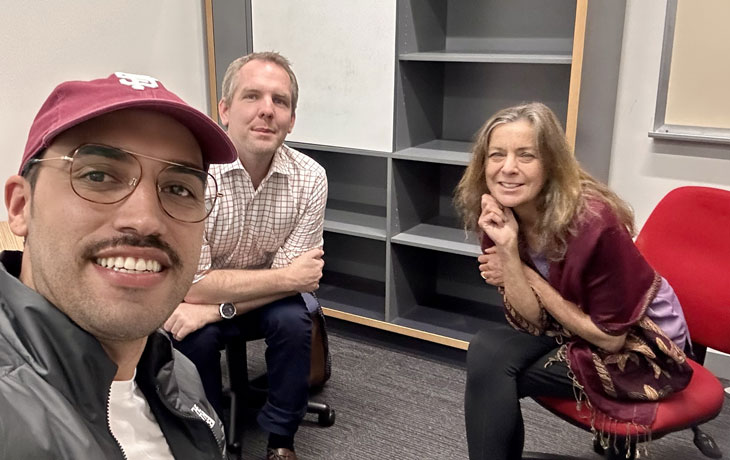
Can you tell us more about this new archaeal group?
Asgard archaea are a very interesting group that has gotten a lot of attention lately because they are the closest microorganisms to eukaryotes. I don’t mean that chronologically, but Asgard archaea and modern eukaryotes share the same common ancestor. That opens a new question about the role these organisms played in the evolution of complex eukaryotic lifeforms—specifically in soil because these microorganisms are typically only found in extreme environments. Asgard archaea specifically were found in a deep-sea hydrothermal vent that scientists thought looked like Loki’s castle. That’s where the name came from, and also where others like Thor archaea, Loki archaea, and Hel archaea are found.
But we found these new archaea—which we’ve named Atabeyarchaeia—in California soil. I didn’t have to go to deep sea vents to find them, which is exciting when you think of them in the context of methanogenesis. Even though they don’t consume or produce methane to the best of our knowledge, they use all the soil substrates that are important for methane. If we know more about these archaea, as well as the greater archaeal community, then we can better understand how to manipulate genomes in a way that has potential implications for addressing climate change.
What is the significance behind the name?
Naming in the field of genomics is hard, but Jill encouraged me to find a goddess from Puerto Rico who we could name the archaea after. I googled and found Atabey, who, in Taino culture, is the goddess of spirituality in soil and water. It was a perfect name, and thankfully, it has been very welcomed in the community. Now we have a Caribbean goddess represented, which is really important to my identity as a scientist and ties to my culture. And as people continue to find more Archaea, they can start using more inclusive nomenclature to expand beyond Nordic gods and goddesses.
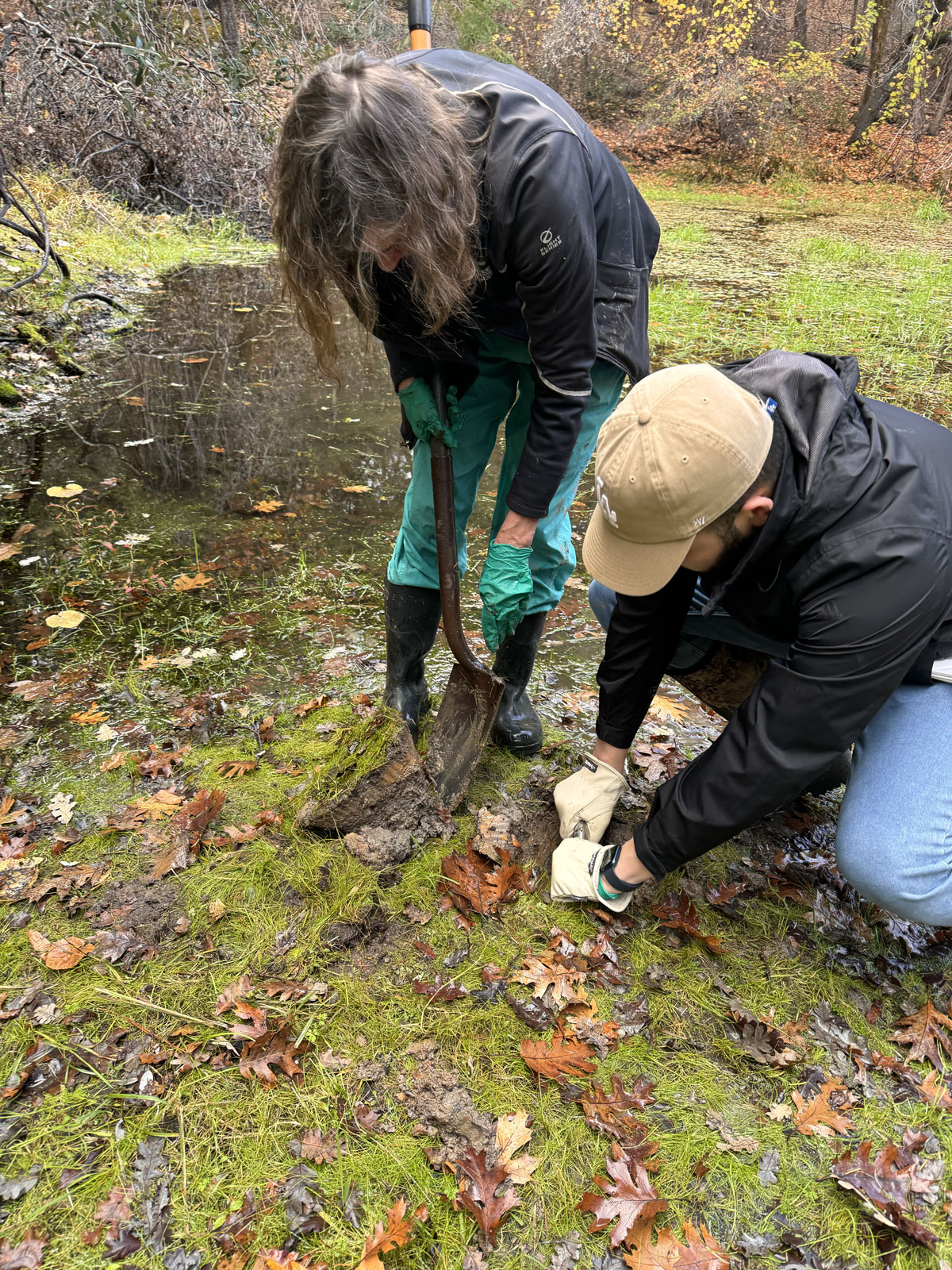
What role do these organisms play in their ecosystems?
One important aspect is new metabolic adaptations in archaea, suggesting they play a role in hydrogen cycling in diverse, oxygenless ecosystems. This was the subject of the Cell paper. We identified hybrid hydrogenase complexes in archaea. These complexes are formed through the fusion of [FeFe] and [NiFe] hydrogenase and have the potential to either produce or consume hydrogen, which is something we believe to be only present in eukaryotes and bacteria. But because we have established this link to Asgard archaea, these hydrogenases could offer an important clue in understanding the evolution or transition from unicellular life to multicellularity. It also suggests that it's possible to find reservoir potential hydrogen in different anaerobic environments. We’re just beginning to understand the basic biology of hydrogen in anaerobic environments, and what we’re doing is an important first step. Once you understand how these microbes are using hydrogen you can start thinking about how to engineer them for different purposes. You can think about it like CRISPR. CRISPR was first discovered in a survey, then we learned more about the basic biology, and now we’re using it in different applications.
The other exciting finding is that up until now, most studies on Asgard archaea have been from the perspective of eukaryogenesis. Our Nature Communications paper on Atabeyarchaeia focuses on the role of the organism within carbon metabolism, particularly with respect to methane. These archaea co-exist with methanogens and use acetate, formate, and hydrogen—three important compounds that are required to produce methane. There is likely some kind of competition with methanogens that we need to better understand.
We also provided three complete reference genomes—two from Atabeyarchaeia and one from Freyarchaeia—which hadn’t been sequenced before. One of the biggest limitations in our field is the incompleteness of genomes. Only three other complete genomes from the same group have been published until now. We hope this will be used as a reference within the community for other studies, specifically as they try to determine whether or not there is any contamination within their samples.
Where do you see your research taking you next?
When Jill first started her lab, having a complete genome—a circular genome—was a big deal. But thanks to advances in technology, there are now hundreds of potentially complete genomes. We're getting to the point in microbiology that recovering a complete genome is no longer a limitation. Our lab and many others are generating massive amounts of data—we are saturated with so many complete genomes that we need first to validate, which is difficult due to the limitation of computational resources.
However, to truly advance the field, we need more collaborations like our partnership with the Greening lab. Only by expressing proteins in organisms and validating them can we definitively prove that the proposed biology is occurring. Another approach is to enrich or isolate these organisms, a process that could take years but is already yielding results. In recent years, we've seen several papers describing new cultures of novel organisms that were previously only known through metagenomics.
Looking ahead, I'm transitioning to a postdoctoral research position where I'll be working to combine ecosystem-scale genomics with biochemistry and structural biology. I'm particularly excited about leveraging emerging artificial intelligence applications, such as generative AI, to guide more effective experimentation. While we now have the power to model and predict structures, the crucial step of laboratory validation remains essential.
This integration of computational predictions with wet-lab validation represents the next frontier in our field, promising to accelerate discoveries and deepen our understanding of microbial life.
Read More
New Research Uncovers Humans’ Closest Microbial Relatives (Innovative Genomics Institute)
Surprises from the hidden world of Asgard archaea (Nature)